Herman Sintim Sigma Xi Lecture
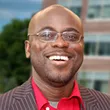
In fall 2016, Sigma Xi Distinguished Lecturer Herman Sintim discussed "bacterial conversation" and how it can be stopped to prevent infections from spreading. Sintim, a native of Ghana and graduate of Oxford University, is the Drug Discovery Professor of Chemistry and Organic Chemistry/Chemical Biology at Purdue University.
This lecture was sponsored by the Swarthmore Chapter of Sigma Xi, the scientific research society, and is designed for a broad, non-specialist audience. He is introduced by Professor of Mathematics Deb Bergstrand, this year's president.
Audio Transcript
Deb Bergstrand: Thank you all for coming. My name is Deb Bergstrand, I'm in the Math-Stat department here at Swarthmore. I'm this year's president of the Swarthmore chapter of Sigma Xi. Before I forget, I just want to remind folks going to the dinner afterwards, it's in the Scheur room. You can just trickle out over thereafter the talk. I think the official start time is six, but given the question and answer and lively discussion, it will have ... We'll get over there shortly before 6:30.
Before I introduce our speaker, I want to give a tiny bit of history of Sigma Xi. This is a research society, a society founded to support and honor research in scientific fields. It was founded in 1886 at Cornell University. The name Sigma Xi comes from the Greek letters Sigma and Xi. It rose from the little motto, I don't know how to pronounce things in Greek, but it's roughly, "Spoudon Xynones" which translates to, "companion's in zealous research", so that's where it came from.
One of the things that I think is great about Sigma Xi, is within a year there were five women elected [inaudible 00:01:17] membership in the society. So yeah, cutting edge there. The sophomore chapter was founded in 1922 and it was the first chapter at a holy undergraduate institution, being sophomore. One of the things that we are able to do with a very nice endowment is to bring distinguished speakers to campus, have lovely dinners and also to cover the first year of membership for senior associate members and first year faculty who become members, and we also offer [inaudible 00:01:48] to appropriate requests from students. It's really great to part of such a lively chapter with such great results. So thank you all for coming to our first event.
I would like to introduce our speaker now, Professor Herman Sintim is from Purdue University. He is the drug discovery professor of chemistry, organic chemistry and chemical biology. You wear many hats. He got his B.S. degree in medical chemistry from the University College London and his doctorate of philosophy in organic chemistry from Oxford University and postdoc's at Oxford, following his degree and then at Stanford University. And began your independent career at the University of Maryland in 2006, only nine short years later, already rises to the rank of core professor, and he was recently moved to Purdue University. And he's on the national Sigma Xi organization list of distinguished lecturer's, which is how we have him here, which is really terrific.
So, we're going to hear about bacterial conversation, what is it, and how can it be stopped to prevent and stop the infection's from spreading. So join me in welcoming your speaker.
Herman Sintim: Okay so I'm going to start by thanking Sigma Xi Swarthmore for the kind invitation. I have really enjoyed my visit so far, this is a really beautiful campus. I am [inaudible 00:03:33] to buildings and green spaces and this is a lovely place to be.
Okay so my hope today is to share with you some of the research that has been done in my lab, but I do have to share that the audience come from broad disciplines and so what I'll do is that I will not go too much into the actual chemistry and biochemistry, but instead I will provide an overview of some of the challenges in developing antimicrobials and hope that by the end of the lecture I at least will be able to convince you that we need a different approach to developing antimicrobials.
So I manage a group of about ten graduate students and also postdoc's at the [inaudible 00:04:33] Purdue University. So I always like to start my slide by reminding us all that we are lucky to be alive in this generation because if we were born maybe, a few centuries ago, life wasn't as good as it is now, right? So we can see here that two hundred million people succumb to measles and I don't think I've actually met anyone who's had measles in the last twenty years, at least, in the western world, right? And that is due to great advances done in medicine and the fact that we are vaccinated.
So we can see here that smallpox also killed a lot of people early on. What is interesting is that it turns out that viral diseases have actually killed more humans than bacterial or other pathogenic diseases, right? But having said that, bacterial also has had this fair share, so for example, the black death. That was a bacterial disease.
When we look at this and [inaudible 00:05:44] more than an error, we must be lucky, but it is only decades ago that we have been told that young soldier's will return from the war and survive all the rummages of war, right? All the injuries, only to succumb to some minor wounds that they sustained after the war. It's because these wounds become infected and about a hundred years ago there were not that many antibiotics, that we take for granted, so just having a minor infection could actually lead to death.
From my culture in Uganda, whenever someone delivers, the first thing that they'd say is that, mother and child are okay. I presume that that came from the fact that several mothers and children do not survive the childbearing experience, due to infections. There's also been instances where people will just step on nails and they will end up having some form of infection, right? So, that will also lead to death.
We really have it so good. We don't have to experience all of these misfortunes. And because we have it so good, we think that this is going to last forever. What I hope to do is to give you a few examples that I try to show that this might not last forever if we don't take any action.
There was a period, about sixty or so years ago that was considered as the golden era of antibiotic discovery and that let to the award, several Nobel prizes, and so the first Nobel prize that actually went to [inaudible 00:07:48] was for the discovery of the antibacterial effect of Prontosil. Today, Prontosil is not considered to be one of the premier antibiotics used in a clinic, but at that time that was actually [inaudible 00:08:06]
Then I hope that many of you know of Penicillin, right? We also take Penicillin's for granted. But that was a sort of hallmark discovery in medicine. The discovery Penicillin actually saved a lot of lives. Once again, a Nobel Prize was given for this certain discovery.
Then finally, in 1952, another Nobel Prize was given for the discovery of yet another antibiotic. So it was a good time. In 1967, so many antibiotic's had been discovered that we all became pompous head and the U.S. surgeon general said that, it is time to close the book of infectious disease. A few Nobel prizes were given, so many drugs were working, and so therefore [inaudible 00:09:13] to tackle other diseases like diabetes, cancer, neurological diseases and infectious diseases were a done deal.
If that is the case then why am I here? [inaudible 00:09:27] Swathmore they didn't get a medal. That the chapter was closed several decades ago, and perhaps the chapter was really not closed. Now, if we look at a graph that shows life expectancy, what we see here is that life expectancy was around the 40's, so I recently turned 40, so if I was probably born one hundred years ago, you might have all come to my funeral maybe a few year ago. Then because, see here that around the 1900's, life expectancy started going up and that actually coincided with advances in medicine. Right, antibiotics are being discovered, antivirals were being discovered and so you can actually see that coordination.
The idea is that if these drugs work as they should and actually discover more drugs than one can then predict that maybe this will go up until it gets to a point that, maybe we'll plateau because no one will live forever even if we come out with an anti-aging pill. That will be the projection. If you look at the U.S. life expectancy, so now this is taking that group of graph, and I tried to concentrate it on the U.S., we can see here that more than a hundred years ago, life expectancy's around 50 years, and then there was a period where it dips back down and that can be explained by the influenza epidemic. Then it continually went up, and so now it is close to 80 so that is good. We live in a good time, we can all, the majority of us are going to grow old and hopefully we're not going to suffer from any misfortune.
It turns out that this great news about life expectancy actually improving is not getting better. We go to trend, it's in the same direction. So we compare life expectancy between people who live in the develop world, which is almost 80 versus [inaudible 00:11:59] of people in the developing world, we see here a discrepancy. The question is that, is it because people who live in the developing world act different, do they have different metabolism, do they have different physiology or could it be a lifestyle? One would even argue that if it was a lifestyle, then our lifestyle actually does not help, right because we've almost become sedentary, we don't move as much. But that is related to the [inaudible 00:12:31] of drugs, antibiotics, antiviral and all of the [inaudible 00:12:37] of modern medicine.
So what this graph tells us, is that it tells us that this increase life expectancy that we have is not really intrinsic to us as human beings, it's actually related to our environment. It's related to the ability of the drugs. If the good drugs that we have stop working then we are going to pay that price, but that price would be more than [inaudible 00:13:08] because remember that those who live in developing nations also still have antibiotics, they just don't have the really good ones. So what that means is that we could move from here all the way to the other. I'm not trying to scare you, I'm going to give you some hard data to actually back this up and then I hope that I will have a call to action that it is our responsibility to actually find this hidden tool, if we don't do something about this.
So let's now look at the antibiotic timeline, that is when an antibiotic was introduced to when a bacterial resistant strain was identified. So resistance is defined as in broad terms as a scenario where the tools that was effective no longer is effective, right? We then have to use a higher dose, you know that, to achieve the same clinical end point. The problem is that if you use a higher dose of a drug than you also have to deal with toxicity issues.
We see here that Penicillin was introduced in the 40's, and by the way, this is from the Center of Disease Control website, so we can actually go and access that. So Penicillin was introduced in the 40's and, oh that's the river, so it was introduced in a clinic in '43 but a resistant was actually already identified. That was terrible. The drug was about to fail. Then, if we look at, so the tetracycline's for example were introduce and if you look at 1950 and compare that to five years later a resistant bacteria strain was identified at the clinic. Now we have a nine year window. There are a few examples, like [inaudible 00:15:08] so [inaudible 00:15:11] is really problematic in our healthcare system. What we can see here that within two years of the drug being developed, a resistant strain was actually seen in the clinic.
Now what we can see here is that around the 70's new antibiotics were not introduced. If you concentrate pompoushead remember the U.S. surgeon general said in '67 that we can close the chapter of antibiotics. So imagine if you were doing research on antibiotics and your research goal was to find a new antibiotic, I don't know where you would get funded, everyone thought that this was a done deal. Everybody tried to remember is that, this could be problematic, that was probably several years later.
Now what is really worrying about this data here, is that these two antibiotic's actually work by a membrane polarization. The amount of mechanism's are different from the [inaudible 00:16:24] antibiotic's. When they were first introduced, the expectation was that because they were no longer using [inaudible 00:16:32] there will be no cross resistance, right, so it would take quite a while for resistance strains to emerge. But what we can see here is that Daptomycin was introduced in 2003 and within two years a resistant strain was identified in a clinic. It looks like two years is becoming the magic number. If it turns out that every time that we introduce a new antibiotic the resistance strain will emerge, then why bother? There will always be a resistant strain.
If we look at the mechanism's of almost all the antibiotics that are currently in clinical use, these are all targeting either, components of the cell wall or [inaudible 00:17:27] cell wall are processes that are important for growth and liability like DNA center cell and [inaudible 00:17:34] so the current strategy is to inhibit growth, okay, that is the current strategy. Leukemia will always have mechanisms to actually inactivate these and so there are so many different mechanism's you can imagine that if you have a drug, that [inaudible 00:18:00] to the bacteria, it will then bind to your target and hope that that binding will lead to some antibacterial effect.
But, there are other ways where some of the antibiotics are also working on the cell wall, but there are so many, oops ... there are so many ways that the bacteria can come out with resistance mechanism's, so for example, the bacteria can have an enzyme that will inactivate a drug or simply, the bacteria can just shoot the drug out. That is the ancient way of getting rid of something, if an intruder gets into your house, guess what that intruder gets, we shoot that intruder away. So there are so many different [inaudible 00:19:01] that a bacteria can actually over express to on that drug.
Now in the U.S. alone, we now have every year over two million cases, illnesses that can be directly attributed to bacterial infections and out of these two million, 23,000 actually result in death. So that is, it's not a very high percentage out of the two million, but if you are one of these 23 it is an extremely high percentage. I always don't like to look percentage because when you're talking about life it doesn't even matter even if it's one. In turns out that there are some killer bacteria that are actually lurking around. So I have an interesting [inaudible 00:19:53] resistant stuff, kills about 11,000, but it effects close to 100,000.
Gonorrhea is a silent disease that normally people are not aware of, but there's almost a quarter of a million Gonorrhea infections in the U.S., so this a sexually transmitted disease that we would have hoped that in this modern era where we have a lot of antibiotics, we would not be scared of diseases like Gonorrhea, but it is actually quite prevalent in our society. Then TD is not very common in the U.S., there's 8,000 infections, but there are over one billion people globally who are carrying the TD. TD actually kills a lot of people in a year. Then of course, there are other bacteria's of course. Now in the U.S. we don't have to worry too much about TD, but if you have been to live in the southern hemispheres or even part of Europe and Asia, TD could be a real problem. We now live in a world where trouble is so easy and so I would actually argue that a problem elsewhere is our problem. So the fact that TD is not common here, doesn't mean that we don't have to worry about it.
We now know that we cannot close the chapter on antibiotics, right we cannot close the chapter on infectious diseases, this is a real problem. It has been estimated that by 2050, deaths from antimicrobial resistant infections will surpass deaths from cancer. I just find this quite astounding, right we're all scared of cancer and we think that bacterial infections are no big deal, but if we look at the rate of resistance to the drugs that we have currently, if we don't have any great foods, then antimicrobial resistance infections would actually become a really huge problem.
We can also see that resistance to common drugs like Penicillin, Tetracycline, and Quinolines are on the rise. So we can see here that for Quinoline, which is a staple antibiotic that is used frequently, around the 1980's there was almost no resistant strains and then it turned out that after 2000 was a huge high there. Several reasons have been put out to account for this increase in resistance for quinoline. Some could be the indiscriminate use of antibiotics, not only in the clinic, but also in any culture. But whatever the reason is, we do have a problem. We have a very useful drug that in a few years, from almost zero to fifteen percent. Now there was a little bit of a dip, but it seems to be on the rise again. If we look at ciprofloxacin, we can also see here that in 1999, we have about 20% of infections were resistant strain and a few years later we are now approaching 70%.
I hope that at this point I have convinced you that antibiotic resistance is a real problem and some of us are going to succumb to bacterial infections due to resistance to these drugs. And so the question is that, why do we need to study to put so much effort and money in finding new antibiotics, right because it doesn't make sense. And say if I was to ask you to predict the number of new antibiotics that are being introduced into the market year in and year out, you would predict that based on that data, there will be several drugs and the trend should grow upwards. You remember by 2050, if we don't have newer drugs that are effective, death from bacterial infection will actually be more than cancer and other diseases. However, if you look at the number of drugs that were approved by the FDA for antibacterial actually, in 1980, so many new [inaudible 00:25:02], the trend is actually going down. We don't have the data for the last few years, but the trend is going down. So this hardly makes sense. It doesn't make sense. You have a problem and newer drugs are actually not being introduced.
But, I'm going to [inaudible 00:25:23] criticize society and how much different companies are saying that they are irresponsible, that is not the case. The reason why there are fewer drugs is that, A, we are running out of targets, right, [inaudible 00:25:38] in fruits, so it's now more difficult to actually come out with a new drug. But another reason is that it is now very expensive to develop a drug. So this shows the number of drug candidates that are nominated by different research groups involving the drug discovery process. So are the candidates nominations state is when people have done their research and they come out with a model pill, it probably works in mice, they show you good signs and they say, okay this could be the drug. So we can see here, that at that stage, a lot of compounds are nominated and then it goes to phase one of [inaudible 00:26:25] trials where we have to test to see if if our compound is toxic to [inaudible 00:26:30]. We can see that by the time it gets to phase one there is a drop off. So a lot of compounds fail because they are toxic to humans.
Phase two is when we'll actually test what they think they see, does this actually work? Again, you see a drop off. By the time you get to phase three you are asking, does this work better than an existing drug? So you can see that my data just falls hard. So the process is highly inefficient and so expensive. So in 1970, it cost about $179 million, right so we're having fewer drugs and the cost is also going up.
Now in the end the pharmaceutical companies are commercial industries. So you should not expect a commercial entity to spend so much resources in an enterprise that actually costs so much. But in addition to that, I also showed you data that whenever you introduce a new antibiotic, within two years there will be resistance. That's when we punish ourselves. [inaudible 00:27:42]
So the question is now, what do we do? This statement has been pedaled around a lot of times, I don't know whether Einstein actually said this, but I just picked it up from some website, they said, "an insanity is when you do the same thing over and over again and you expect different results." Right, so we have been developing new molecules that kill bacteria. That's what we've been doing over decades. Then what happens is that two years later, we then get resistance and we go back to the same problem and we get resistance and actually I would really say, I would really modify this and say that, extreme insanity is when you do the same thing over and over again and each cycle becomes more expensive. You then expect to have a different result.
It turns out that one of the primary reasons that bacteria become resistant to antibiotics, in addition to the molecular mechanism's that I have described to you, is because the majority of the antibiotics that are being introduced into the clinic were developed against planktonic bacteria. In the past chemist's would make molecules and grow bacteria in the lab in a test tube, they add the molecules to the bacteria and then they look for molecules that kill the bacteria. Free floating bacteria. But it turns out that, bacteria spend a lot of their time in a state called a biofilm state. When bacteria are approach a surface and there are so many surfaces around. They then attach to that surface and then as more bacteria join to form micro colonies and then they form these biofilm states, which are [inaudible 00:29:46] of bacteria, sometimes fungi, they secrete polysaccharides, DNA proteins, it almost becomes like a castle, where bacteria is embedded in these biofilm's.
It turns out that in the biofilm state, bacteria can be a thousand times more resistant to antibiotics because we are developing these drugs against several free floating bacteria, but sometimes [inaudible 00:30:16] state. So, what it means is that we need to come out of the way to find molecules that can actually tackle biofilm bacteria. Sometimes in the biofilm state the metabolism of the bacteria is also lower than in a planktonic state, but these molecules are [inaudible 00:30:34] grow from [inaudible 00:30:36] processes. There is almost like a disconnect between what is actually required to deal with bacteria in a biofilm than in the free floating.
If we can understand the biofilm process, if we understand and we collect details of bacteria social engagement, how do they form biofilms, then maybe we will be able to target new processes that come up with newer entities of drugs that could actually tackle bacteria. So we might group, we actually welcome to different aspects of bacteria signal and I won't go into too minor details, but we will call it a process called [inaudible 00:31:22] where [inaudible 00:31:23] actually cycling bacteria to form [inaudible 00:31:30] to second that energy. These molecules actually regulates the biofilm [inaudible 00:31:35].
So the understanding is that if we can identify the proteins and the receptors that are involved in this process than we might be able to tackle biofilm bacteria. When bacteria also effects humans, so this is the whole cell. There are also processes that also evolve [inaudible 00:31:52] so this has become a very attractive target to now figure out or identify new anti-biofilm agents. But remember, that if we can have an anti-biofilm agent, than we might be able to solve the bacteria resistance problem, right, because the two actually makes.
So, it is now known that when this molecule Cyclic Dinucleotide concentration groups suck in bacteria, bacteria forms biofilm, and when the concentration goes down bacteria can actually be spared from the biofilm. It's become a very critical process in bacteria.
But, in addition to the processes inside bacteria, in the biofilm, bacteria also connects to other bacteria and they talk to each other with small molecules. These small molecules shrink outside the processes that lead to biofilm formation. So you can see that the picture is not entirely that complicated. There are molecules outside, so these are [inaudible 00:32:57] produced by different bacteria, it's almost like a language signal in molecules that are outside and they bind to receptors that are on the surface in here, or on the cytoplasm, right? The bacteria is also sensing food and other environmental factors. Then inside there are also so many signaling pathway's. I did briefly talk about cyclic di-GMP pathway's here in the middle of the muscle regulator and even the cyclic di-GMP pathway's even more complicated.
We can see here that there are so many different pathways. So if you're going to develop a molecule, which one do you go for? It's almost like taking a bet, right? You've got to choose the right pathway because if you don't choose a muscle regulator bacteria has come out with a redundance or [inaudible 00:33:47] of taking care of this stress. It also [inaudible 00:33:57] bacteria human cells, there are so many processes and [inaudible 00:34:00] there are receptor [inaudible 00:34:01] processes, right the process is actually quite complex, but if we can understand those than we might be able to cover a newer generation.
So I now return to the title of this talk, what is is Quorum sensing? So it turns out that as bacterial population grows up, the small molecules that will secrete into the environment will also go out, right? So the ability, oops, this is going to fast. So if bacteria population is related to the concentration of a small molecule than one can argue that by sensing the fluctuations in these certain molecules bacteria will at least be able to sense population density and then coordinate behaviors that are going to be beneficial. Now, I must say that there are others who strongly oppose this hypothesis, who don't believe that bacteria actually coordinate behaviors per se, that quorum sensing is just the after effect of metabolism. So there are two views. Some believe this is a social phenomenon, others just believe that it is metabolic by product. But the perspective of which [inaudible 00:35:20] is operated, we know that as a population grows up, the concentration of the molecules in the solution would also go up and there are consequences.
As a chemist, I am interested in the types of molecules and the types of receptors that actually bind to these molecules because once we understand these processes than we might be able to use other molecules like [inaudible 00:35:46] process. So some of the molecules called AI-1 which is a homoserine lactone. For those of you with chemistry background, you will immediately realize that this is going to be unstable to hydrolysis, so why would a system use a communicating molecule that is unstable? It makes perfect sense, because language should be temporal. You don't want a molecule that will exist forever. So the ability for this to actually also degrade [inaudible 00:36:22] of the language. [inaudible 00:36:26] already beginning to see diversity in the signaling and then some [inaudible 00:36:32] and some [inaudible 00:36:38] also use what we call AI-2, so AI-2 was [inaudible 00:36:41] taking a very beautiful [inaudible 00:36:45] by the [inaudible 00:36:49] here because you have, one of your professors was actually one of the leading authors on some of the most [inaudible 00:36:57] that I try to [inaudible 00:37:01] of AI-2.
I have always been fascinated by AI-2, the reason by I've been fascinated by AI-2 is that because seeing that single molecule can exist in so many different forms and giving the presence of certain metals or certain elements in the environment can even shake the equilibrium. To me AI-2 is a beautiful language because not only can it exist in different forms, the molecule itself can even sense its environment, to me that is very beautiful.
It turns out that the pathways have been oxidated by several groups. As I said I'm not going to go too much into the pathways, I just want to give a general overview. We can see here that different molecules bind to different receptors and this leads to different transitional responses, some could be [inaudible 00:38:01] borrow information or [inaudible 00:38:04] etc. The idea is that if we can understand them and identify the [inaudible 00:38:12] than we can use drugs or [inaudible 00:38:14] to block these and hopefully you will be able to stop biofilm formation. [inaudible 00:38:20] of Ai-2.
Now I would argue that one of the seminal papers that has been published in the quorum sensing field was a paper by a chap called Suda. So what Suda did was that he took, he looked at the natural AI-1 molecule which promoted bacterial biofilm and he made an argument that if he was to make an analog that was similar but different than that analog might bind to the same receptors, but instead of promoting quorum sensing behaviors in biofilm information, maybe that analog will inhibit that process. So in 2003 he basically made a molecule, you can see here that this molecule looks almost like this, just that the head group is different. He showed that when you add this synthetic compound, the synthetic compound can compete with the natural compound. So here you grow bacteria without the quorum sensing molecules bacteria doesn't form biofilm, but in the presence of this quorum sensing molecule it forms the biofilm, so they agree this [inaudible 00:39:33]. Then when he added this molecule, he was able to use biofilm.
This was a seminal paper because now there was direct proof that you could actually use chemistry to intersect this signal, this bacteria conversation. This is regarded as one of the most quantum papers. Now earlier I had mentioned that the AI-2 pathway is one of the most beautiful language because it exists in so many different forms. Once again, Besler has done some really beautiful work where she showed that AI-2 can also be internalized, [inaudible 00:40:12] and then once it gets internalized it gets [inaudible 00:40:16] form binds to a represser. That then gets de-repressed and then that leads to the expression of different genes.
[inaudible 00:40:25] worked out and is now turning on other pathways, a little bit more complicated, E coli doesn't make AI-1, but it concerns AI-1 and, it turns out that E coli can also integrate sugar and [inaudible 00:40:39] signal and also [inaudible 00:40:42], so very intricate and nice signal and pathway. I like signal and pathway's because it gives so many opportunities to actually assume different levels.
The question then was, following Suda's signal way. Could we also make AI-2 analogs that would also intersect with AI-2 signaling. Yes?
Speaker 3: Are these pathways going to be different for different bacteria or is it more of a general process?
Herman Sintim: That is an excellent question. It's a yes and no. There are some bacteria that will use similar pathways, so for example, the AI-2 pathway equalizes similar, but different [inaudible 00:41:30] for example. That also brings separate challenges. That you're coming out with a molecule that can intersect AI-2 and AI-1 signaling in one bacteria, but it might not affect other bacteria, and in nature biofilm's are polymicrobial. So you could stop one bacteria from not forming the biofilm, but the rest are not listening to your instructions. So this is a complicated machine.
The idea here was that we could also use chemistry, just like in the AI-1 case, to also intersect the signaling. So several groups, including my group, [inaudible 00:42:14] a beautiful work he's made several AI-2 analog's, he came out with a very nice synthesis in 2004 and then in 2009 he came out with an alternative synthesis. I'm not going to spend my time talking about which synthesis is better, I would say they are complimentary, they allow one to make different analogs.
So because he came another synthesis we were able to make so many different analogs of the natural signaling molecule, then went and we tested to see if our analog could naturally affect quorum sensing in e coli. Here, quorum sensing is off because there is no AI-2 in e coli, AI-2 is internalized, it gets [inaudible 00:43:07], it binds with [inaudible 00:43:07]. So here is the [inaudible 00:43:12] chain reaction, very, very important. AI-2 has [inaudible 00:43:16] here. And so, what we have done over the years, is that we have collaborated with others to solve the structure of [inaudible 00:43:28] in complex with AI-2 because if we had a looser structure than it would allow us to be able to give [inaudible 00:43:35] the analog's that they actually do. So this was done in collaboration with a new group at [inaudible 00:43:41] at [inaudible 00:43:43] science institute.
We then tested our analog's and we used e coli that have a [inaudible 00:43:55] protein that was controlled by LsrR. And so the idea here is that the naturally signaling molecule will bind to the repressor, repressor comes off, [inaudible 00:44:04] we can see that the [inaudible 00:44:04] molecule is working. Interestingly, molecules that look like the natural molecule, but have some slight modification, could compete with a naturally signaling molecule and block signal. So see, This is similar to what Suda did. A theme that actually measures that you can look at a natural signaling system, make analog's and do small changes and this would then go and compete with the natural molecules and actually give you an opposite phenotype.
Now, AI-2 is evolving biofilm formation in e coli. If we have an AI-2 like molecule that could compete with a natural molecule than there is a possibility that this could also have anti-biofilm effect. So in [inaudible 00:44:54] we actually test his hypothesis and we were able to show that when you grow e coli in the presence of the natural AI-2, it forms robust biofilms, right, the color is visible, every time we go to biofilm we stain the biofilms, we stain the carbohydrates, proteins, etc. The more color you see means that it has more biofilm. Then, when we added our molecules, I wanted to focus here, and if you are a chemist you can see that there is just a slight modification, we could actually compete with a natural molecule and reduce biofilm. So the principle works, you can look at a natural signaling system and come up with molecules that will compete in a natural signaling system and then that will then lead to anti-biofilm.
Now here is a problem with biofilm [inaudible 00:45:47] molecules. If you have an infection and if bacteria has formed biofilm, it's inside the body. If your molecule gives these spaces that bacteria all you are doing is that you are just taking a problem from one site to the other site. The bacteria [inaudible 00:46:10] grows to form biofilm elsewhere. So what we really want is that we want to dispense out and then kill it. Then what we then did is that we combined our molecule with an antibiotic that doesn't really work well, so gentomyocin is a gentle drug, when you brew e coli biofilm's and you add them to [inaudible 00:46:28] works, but not that great. When we then add our anti- we can actually get better reaction to the biofilm.
Now pseudomonas is an interesting pathogen, it doesn't make the AI-2, but it senses AI-2. There are some bacteria, they don't make certain molecules, but they can also [inaudible 00:46:48] because of the environment you also have to say this about molecules too. So it turns out that with pseudomonas we also have an analog so that we can here that gentomyocin does not work on pseudomonas biofilm. If you add gentomyocin to pseudomonas it's almost the same. We add our AI-2 analog and can actually predict that.
After we showed that AI-2 can synergize with antibiotics, I have a colleague, a former [inaudible 00:47:22] who is interested in clearing bacterial biofilms using electric signals. So it is known that electric channel you can actually use electricity to disperse biofilms. You might ask, why would you want to do that? But you can imagine if you have some form of [inaudible 00:47:39] where biofilms are going to form, if you can have a situation where you change the current, the current will disperse the biofilm, that would be a neat strategy. So we ask, could we use our small molecules to synergize this bio-electric effect. What we did was we collaborated with our collaborator, so this is an experiment done in the microfilled chamber, so here you have bacteria biofilm, you can generate some electrical current and the bacteria will come off. So the question is, can we synergize to disperse the biofilm and it turns out that yes, we can do that, so this is A is bacteria grown with that electric current. If you then treat the bacteria biofilm with electric current you just get a little bitty space out, that is B. If you treat bacteria biofilm with that molecule you get more disperse our with electric current, but if you combine the two you really get a lot of this stuff off.
You might ask, why do we still have some blue color, is it not bacteria anymore? Is that when a bacteria leave the biofilm they leave polysaccharides behind, so when we stain we're going to see a polysaccharides stain. So that combination was you can actually combine our molecule with biofilm. Right, so controlled electric filled our analog, a combination, and you can see here better, bacteria biofilm, you have electrical current, you have just a little, while I would say that this is more significant. You add our analog, you get reduction, but you add both, you see synergy.
I hope that I have been able to convince you that you can [inaudible 00:49:25] signaling pathway, identify the players, identify the signaling molecules and make molecules that are similar to the signaling molecules and they will actually be able to compete and lead to very interesting field science.
Now I did mention that peptides are also signaling molecules. So [inaudible 00:49:47] group are this concerned, they have also been doing some really amazing work where she has also shown that you can also take a similar peptide and make an analog and that analog can compete and also stop biofilm formation to start. So it looks like this is becoming a recurring theme. In respective of a signaling molecule, one can replace an analog that can naturally compete.
In the last maybe five minutes or so, I want to talk briefly about [inaudible 00:50:25]. I could talk about quorum sensing outside the cell and then what is inside. If you can target both than you will be able to stop bacteria biofilm formation. So this molecule is called, [inaudible 00:50:36] it was discovered in the late 80's and the scientific community basically ignored this molecule for almost two decades. There was a very nice mid term paper published in '87 that showed that in some bacteria, DTP is committed the psychic form and the psychic form is actually evolving some of those synthesis. Right, '87, 20 years later, there was a seminal biophomatics paper that showed that a lot of bacteria have the enzymes that make [inaudible 00:51:17]. That was when people started asking, are these doing something? Because bacteria is not going to [inaudible 00:51:24] just out of nothing, right? There has to be at least some genes that appear to not have uses at the moment, probably because we don't really know what it do, but it doesn't make sense to put so much effort and currency in it if there are no use to it.
Since then, it has been discovered that not only is [inaudible 00:51:45] found in bacteria but [inaudible 00:51:47] is also found, so if you look at difference, '85-'87, the next one was funded in 2008 and then after 2012, there was another molecule in 2012, there was also another molecule found. This has become a very hot topic in microbiology. The understanding now is that the quorum sensing happens outside some of the molecules, but inside bacteria you have [inaudible 00:52:13] and that leads to biofilm formation. It also turns out that when bacteria, such a TD does get internalized into whole cells like macrophages, once they eat a microphage some of the TD gets out the whole sense is that it's a bigger signal, the whole also produces or makes [inaudible 00:52:36] that then grows food leading to immunity response and then of course takes care of the bacteria.